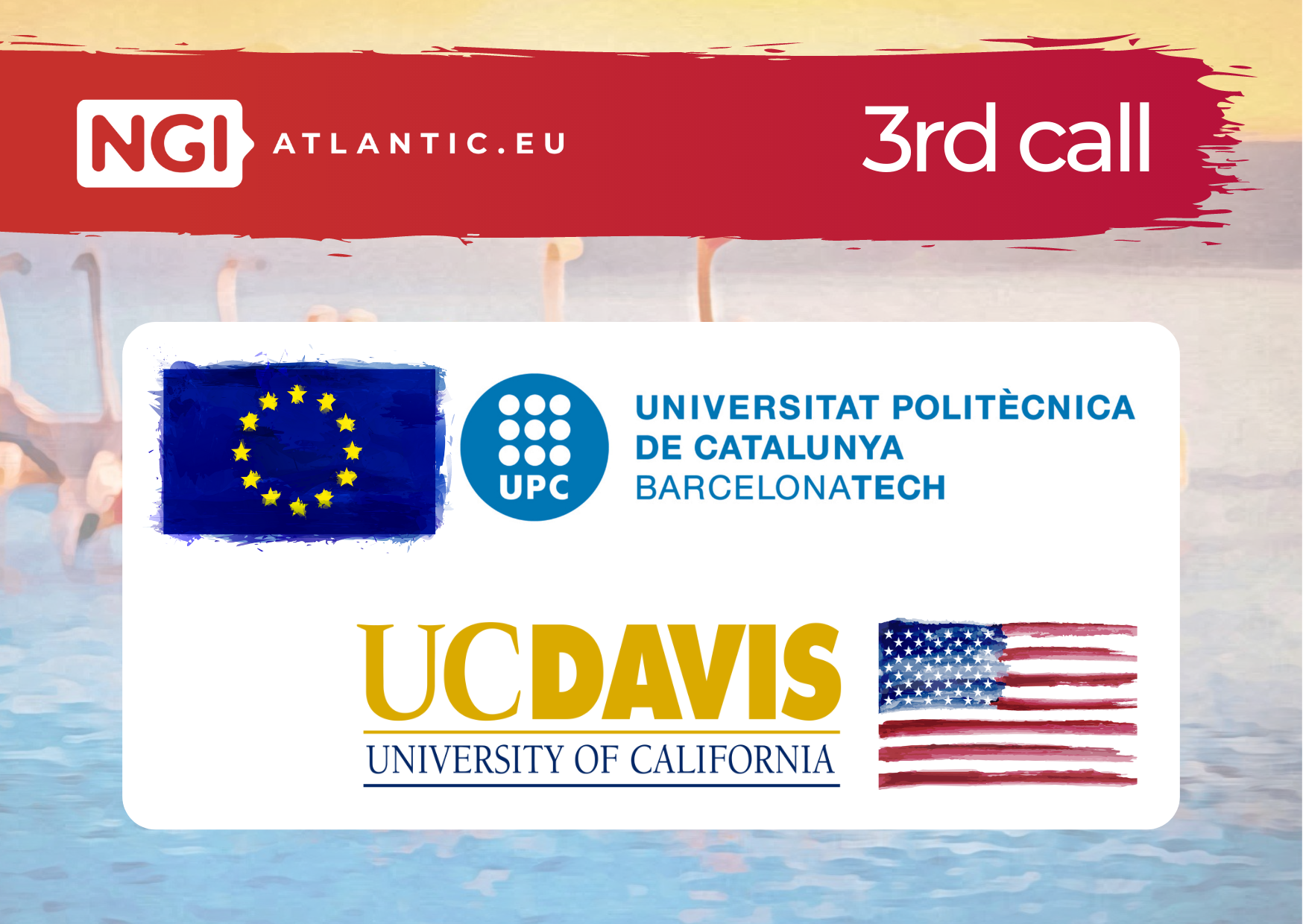
Project Coordinator (EU) :
UNIVERSITAT POLITECNICA DE CATALUNYACountry of the EU Coordinator :
SpainOrganisation Type :
AcademiaProject participants :
EU:
Prof. Luis Velasco, MSc. in Physics in 1993 from Universidad Politécnica Madrid PhD in 2009 from UPC.
Prof. Jaume Comellas, Telecom Engineering in 1993 from UPC, PhD in 1999 from UPC.
Prof. Gabriel Junyent, Telecom Engineering in 1973 from UPM, PhD in 1979 from UPC.
Dr. Marc Ruiz, MSc in Statistics and Operations Research in 2009 from UPC, PhD in 2012 from UPC.
Seyed Morteza Ahmadian, BSc. in Information Technology engineering in 2009 from Razi University of Zanjan/Iran.
US:
Prof. S.J.Ben Yoo, MSc. in Electrical Engineering, 1986, Stanford, CA, USA, PhD in Electrical Engineering, 1991, Stanford, CA, USA.
Dr. Roberto Proietti, MSc. in Telecommunication Engineering, 2004, University of Pisa, Italy.
Dr. Sandeep Kumar Singh, MSc. in Electrical Engineering in 2014 from Indian Institute of Technology Madras, India PhD in Computer and Network Engineering from Tech. University of Braunschweig, Germany.
Mehmat Berkay On, BSc. in Electrical and Electronics Engineering in 2018 from Bilkent University Ankara/Turkey MSc. in Electrical and Computer Engineering in 2020 from UCDavis, Davis CA/USA.
State of US partner :
CaliforniaStarting date :
Experimental Assessment of Fast Quantum Key Distribution
Experiment description
QKD relies on properties of quantum mechanics, which enable eavesdropping detection and guarantees the security of the key. Among QKD systems, polarization encoded QKD has been successfully tested in laboratory experiments and recently demonstrated in closed environments [5]-[7]. The main drawback of QKD is its high cost, which comes, among others, from:
- the requirements for quantum transmitters and receivers;
- the need of carefully selecting the fibers supporting the quantum channel to minimize the environmental effects that could dramatically change the polarization state of pho-tons.
The expected impact of the Fast QKD method is in facilitating the deployment of QKD systems to provide long-term data protection in a post-quantum world, by reducing its cost. On the one hand, some hardware specifications of the QTx can be relaxed as SOP imperfections can be corrected by the QRx, whereas on the other, the hardware design of the QRx can be simplified and rely on software.
Impacts :
Current research efforts are focused on demonstrating such performance in real and challenging scenarios, including aerial cables, where QKD transmission might be severely affected by weather conditions (e.g., high wind) that stresses optical fibers, increase qBER, and eventually reduce the effective key exchange rate, e.g., from Mb/s to Kb/s or even b/s, as shown in [8].
This project targets at confirming such performance, with the proposed experiments, which will allow keeping its performance when aerial cables are used. In consequence, this project will noticeably contribute to the identified impacts of the NGI initiative.
Impact 1
With the identified tests, the EU and US team have developed unprecedented collaboration in these aspects. This project gave the opportunity to the EU and US teams to gain huge experience on Quantum com- munications related experiments. In particular, during the tests we found several prob- lems coming from reusing old equipment; in particular problems with the calibration of the EPC that prevented to follow the tests as originally planned.
Impact 2
Where EU - US collaboration will impact future research programmes. Open QKD-related experimental data is nowadays very scarce. Therefore, we expect that the open data-set made available by the project will be very useful for future research and innovation actions.
Impact 3
Where a joint demonstrator is being built and can be used in the future to contribute to the standardization of the QKD technology, in particular for its use in aerial optical cables.
Impact 4
Where EU - US top researchers working in this project are creating the basis of an ecosystem for collaborating on the evolution of the Internet. Again, the availability of the open data-set made available to the research community will have high impact as it will promote new ideas and accelerate the evolution of the Internet.
Results :
This experiment focused on generating results from 5 sub-experiments.
Verifying the Single Photon Emitter (SPE)
Description: To build a single photon emitter (SPE) for QTx, we attenuated a C-band distributed feedback laser. As shown in Figure 3 (in D3) the operating wavelength of the laser is 1551.74nm and the minimum power output of the laser unit is -10 dBm. To reach a range of 1000 photon/second level, we placed one fixed attenuator with 40 dB attenuation. At 1550 nm, 1 mW (0 dBm) power approximately corresponds to 1016 photon/second. To flexibly tune the photon rate, a variable optical attenuator (VOA) was used. VOA operation range is 0 to 60 dB attenuation. The insertion loss of the VOA unit was recorded as 4 dB experimentally. We ran the VOA above in the 15 dB attenuation range. In this way, even without the added insertion loss of the units, the SPE cannot exceed -65dBm optical output which is the maximum optical input power for the single photon detectors (SPD).
Conclusion: The results show that with a VOA attenuation level of 49 dB, we could have 1,000 photons counted in the photon counter. So, buckets of 1000 photons can be generated with these configurations settings in the SPE (QTx) and Photon counters (QRx): VOA= 49db attenuation (total attenuation=49 + 40 + 10 = 99 dB), integration time in PCs = 0.1 seconds.
Verifying the Polarization Encoder, fiber, PBS and SPDs
Description: The QTx sends predefined sequences of polarized photons and the PCs should count them correctly. The EPC must be tuned to not introduce any polarization effect. The aim of this verification is to ensure that emitted polarized single photons by the SPE can be encoded and received in any polarization state.
Conclusion: Although the results are not as precise as the expected results, they confirm the correct generation of photons with desired polarization states in the QTx as well as the correct installation of the fiber between QTx and QRx, PBS and PCs in the QRx. The misalignment is related to the fact that we are visually encoding the polarization states with the MPC. To produce more precise results, we would need an accurate polarimeter (the UCD team has been trying to acquire one but there have been some delays in the procurement process).
Verifying the SOP estimation function
Description: The QTx will emulate SOP effects by sending predefined sequences of polarized photons that would correspond to the desired SOP distortion. A function in the Fast QKD software will recognize the distortion using an MPC before the PCs. The aim of this verification is to ensure
that we are able to estimate the distortion amplitude arising in the quantum channel.
Conclusion: We defined the aforementioned experiment to be performed with the MPC, which cannot precisely change the SOP and it is adjusted visually. Considering this imperfection, we approximately estimated the polarization movement in the MPC using the SOP estimation function. In conclusion, these preliminary results support the SOP estimation function verification.
Stabilized photon proportions
Description: As we showed earlier in verifying the SOP estimation function section, the function needs three axis measurements to identify the polarization state of receiving photons. But we need to know how many photons are adequate for a precise estimation. To tackle this question, we define an experiment to see when we can count on the measurements.
Conclusion: As we can see in the results, photon counts’ proportion was relatively stable with 10000 counted photons, and as a result 10,000 photons are adequate for each qBER estimation in SOP measurement.
Verifying the SOP distortion compensation
Description: This key and final experiment verifies our fast QKD method, and it contains all components that are verified before. QTx sends predefined distorted photons in buckets. The QRx firstly estimates the distortion using the SOP estimation function and secondly compensates for the distortion.
Conclusion:
We can conclude that our novel approach can compensate for not only the intentional distortion in QTx (which represents a distortion happening in the fiber in real scenarios) but also offsets around the EPC. Therefore, the obtained results support the novel SOP distortion compensation method in true polarization encoded QKD, and enable the proposed fast QKD implementation.
Future Plan :
Performed experiments show how delicate the testbed and equipment are. It illustrates the gap between theoretical simulation and experimental setup used for implementing QKD protocols. This observation motivates the need to consider every imperfection in experimental setups and opens up the opportunity to use digital twins, e.g., based on simulation models, to be able to compensate for the imperfections and distortions and as a result to increase the key exchange rate in challenging environments where QKD protocols have been implemented. Moreover, using aforementioned techniques and models, we will be capable of faster and more safe eavesdropping detection procedures.
In addition to experimental data obtained in this project, more data that have been collected from experiments carried out in collaboration with Telefonica in their MadQCI, a permanent Quantum Communications Infrastructure for experimentation located in Madrid. Because Telefónica’s MadQCI is a production network, useful data have been collected to complement the lab experiments performed in this project and has been used to develop the aforementioned Quantum Digital Twin (QDT).
However, the algorithms in the QDT for optimal configuration and eavesdropping detection cannot be evaluated in the MadQCI infrastructure precisely because it is a production network. Therefore, such algorithms have been evaluated with an ad-hoc simulation environment, which consists of: i) a SOP event generator; ii) a DV-QKD channel simulator; and iii) an authentication channel for key distillation. The results show that the proposed DV-QDT makes DV-QKD systems more robust as it reduces qBER over 50%, duplicates secret key exchange rate under stable SOP conditions, and provides accurate eavesdropping detection.